Powerful supercomputers to uncover the mechanism that activates cell mutations found in around 50 percent of melanomas. Molecular dynamics simulations on TACC's Stampede2 supercomputer tested the stability of the structure of the B-Raf complex, which, when mutated, is linked to skin cancer.
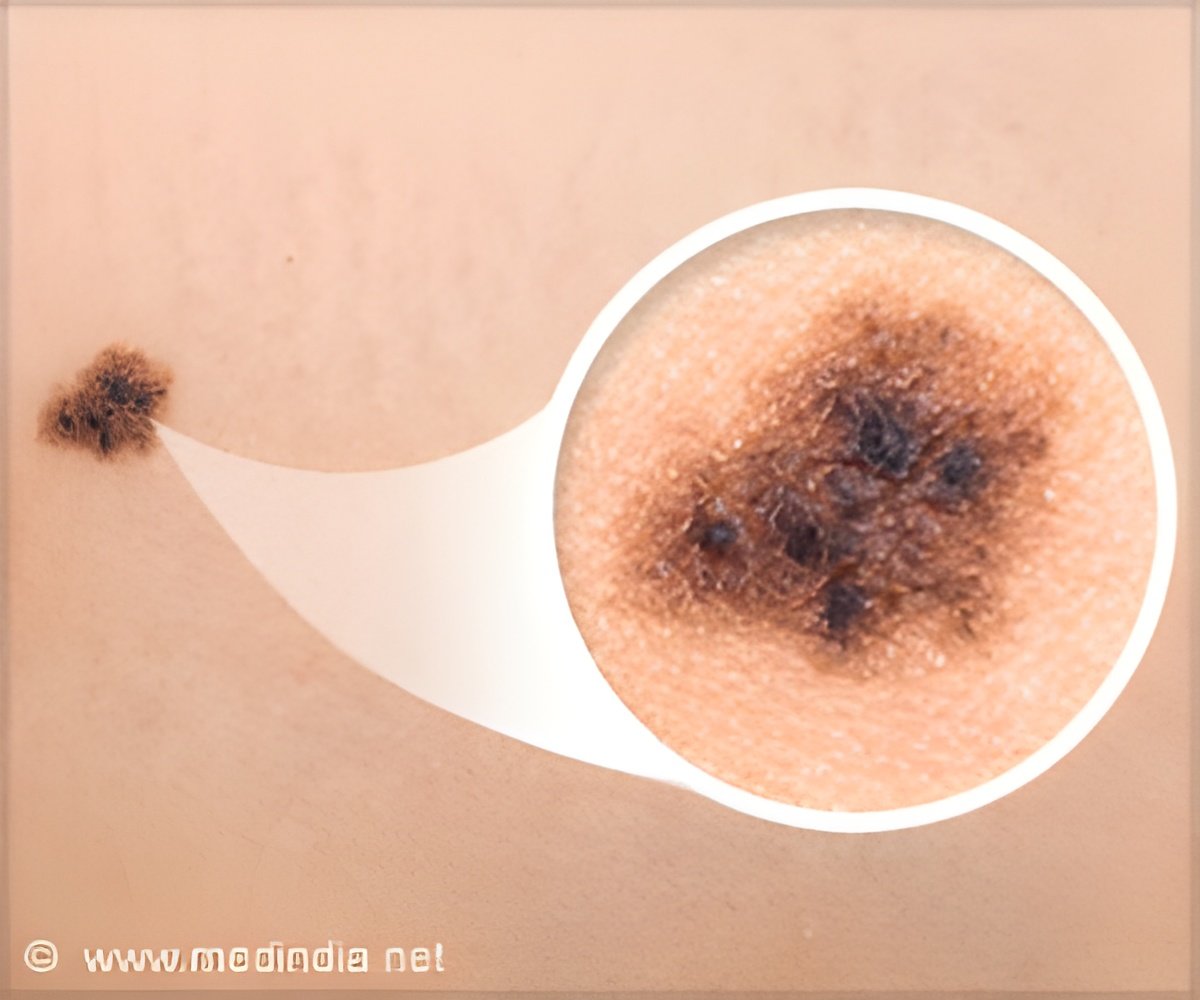
‘Nearly 50 percent of melanomas have a specific single mutation on B-Raf, known as the valine 600 residue to glutamate (V600E).
’
Read More..
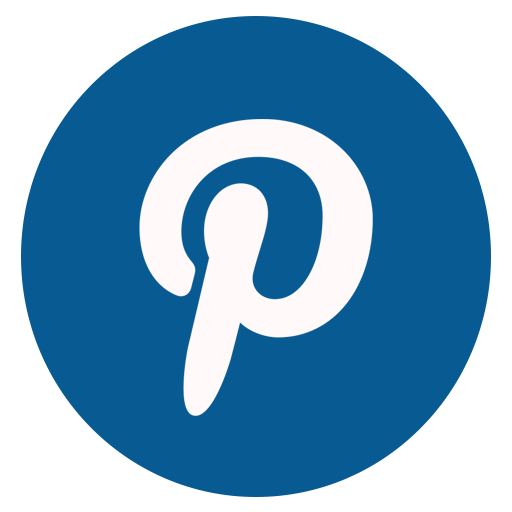
In 2002, scientists found a link between skin cancer and mutations of B-Raf (Rapidly Accelerated Fibrosarcoma) kinase, a protein that's part of the signal chain that starts outside the cell and goes inside to direct cell growth. This signal pathway, called the Ras/Raf/Mek/Erk kinase pathway, is important for cancer research, which seeks to understand out of control cell growth. Read More..
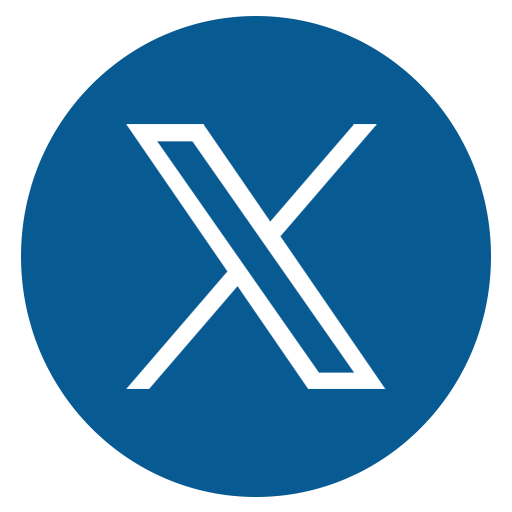
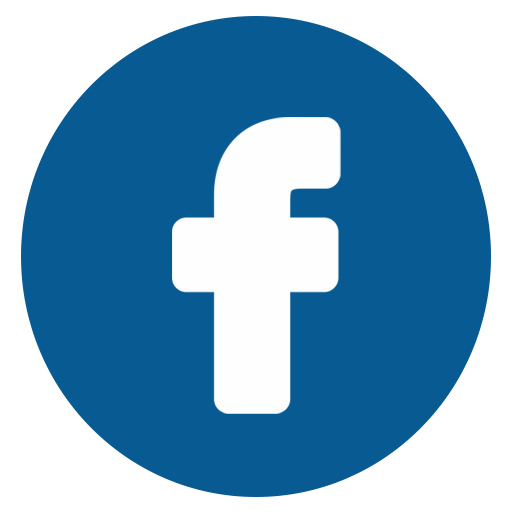
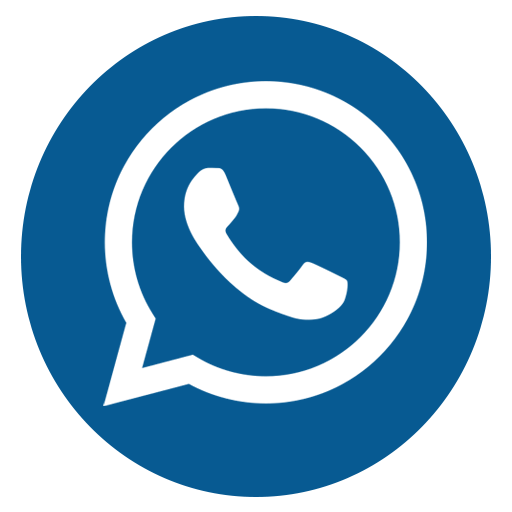
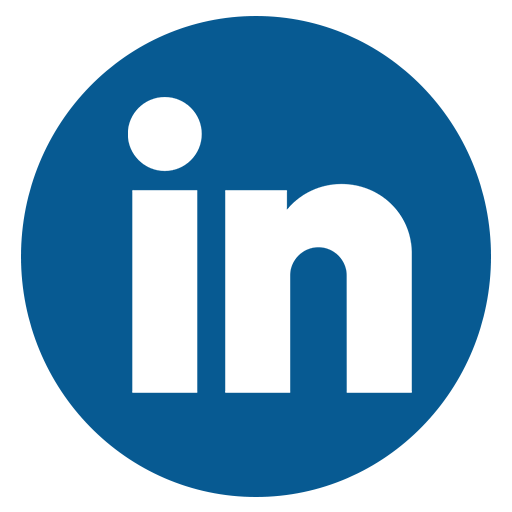
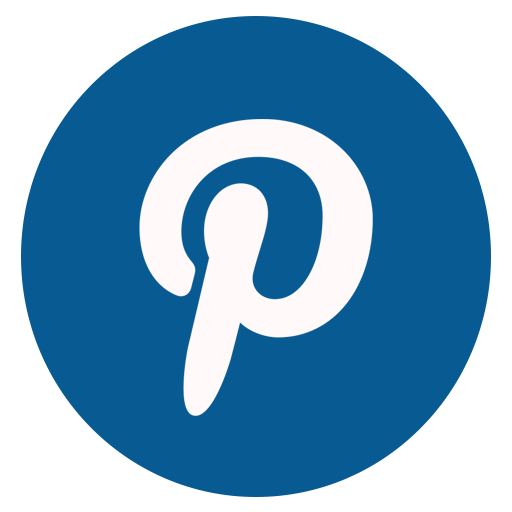
B-Raf V600E thus became an important drug target, and specific inhibitors of the mutant were developed in the following years. The drugs inhibited the mutant, but something strange happened. Paradoxically, quieting the mutant had a downside. It activated the un-mutated, wild-type B-Raf protein kinases, which again triggered melanoma.
"With this background, we worked on studying the structure of this important protein, B-Raf," said Yasushi Kondo, a postdoctoral researcher in the John Kuriyan Lab at UC Berkeley. Kondo is the co-author of an October 2019 study in the journal Science that determined the structure of the complex of proteins that make up B-Raf and also found how the paradoxical B-Raf activation happens.
"We aimed to study the more native-like state of the protein to understand how it's regulated in the cells because most of the studies have been focused on the isolated kinase domain and how the drugs bind to the kinase domain." Kondo said.
The full-length B-Raf protein is made of several domains linked by disordered regions, something too unwieldy for scientists to yet image. Kondo's technique was to use intein chemistry to make smaller fragments, then stitch them up to get the full structure.
Advertisement
Kondo's group used cryo-electron microscopy (cryo-EM) to determine the structure of the B-Raf 14-3-3 complex, basically cryogenically freezing the protein complex, which kept it in a chemically-active, near-natural environment. Next, they flashed it with electron beams to obtain thousands of 'freeze frames.' They sifted out background noise and reconstructed three-dimensional density maps that showed previously unknown details in the shape of the molecule. And for proteins, form follows function.
Advertisement
Detailed analysis of the asymmetrical B-Raf 14-3-3 complex structure showed another unexpected structural feature, described as the distal tail segment, DTS for short, of one B-Raf molecule. Kondo said the tail of one is bound to the active site of the other, blocking its activity by competing with ATP binding. The blocked B-Raf molecule is stabilized in the active conformation. "We interpreted this structure that this blocked B-Raf molecule functions as an activator and stabilized the other B-Raf receiver through the dimer interface," Kondo said.
Curiously enough, the authors compare the B-Raf dimer to the Chinese yin-yang circular symbol of interconnected opposites joined at the tail. "From looking at the subject, it's very clear that one is not capable of phosphorylating the downstream molecule, which is necessary for cell growth. The other molecule is clearly the one to do the job. In this set of two molecules, we clearly see one is doing the supporting job, and the other one is doing the actual work. It really does look like Yin and Yang in this B-Raf 14-3-3 complex we solved," Kondo said.
Looks, though, can be deceiving. Scientists used computer simulations to help verify that they were really onto something. "We ran molecular dynamics simulations of this complex of the B-Raf dimer bound to a 14-3-3 dimer to test the stability of the asymmetric conformation," said study co-author Deepti Karandur, also a postdoctoral researcher at the John Kuriyan Lab of UC Berkeley; she's also a postdoctoral fellow at the Howard Hughes Medical Institute. "We didn't know why the conformation was asymmetric, or what role it played in maintaining the active state of the enzyme," Karandur said.
They started the simulations using the structure that Kondo had solved by cryo-EM, with the DTS segment running from one kinase into the active site of the other. Then they ran a second set of simulations with the DTS segment removed.
"What we found was that in the system without the distal tail segment, the entire complex is not stable," Karandur explained. "The kinase domains move with respect to the scaffolding, the 14-3-3 dimer. In one of our simulations, the dimer state of B-Raf itself, which experiments have shown is necessary to maintain the active state of this kinase, it fell apart, indicating that this distal tail segment, DTS, is necessary to actually maintain this complex in this asymmetric conformation, which in turn is necessary to maintain the kinase dimer in the stable asymmetric dimer active state."
One of the main results of the study was finding the mechanism of action that switches on the B-Raf kinase complex of two B-Raf kinases and two 14-3-3 scaffolding proteins, where on B-Raf kinase is the activator, and the other is the receiver.
"The tail of the receiver molecule is inside the active site of the activator, so the activator cannot work as an enzyme," Kondo said. "Instead, the activator molecule stabilizes the active conformation of the receiver molecule. The 14-3-3 scaffold protein facilitates this arrangement so that the tail insertion only happens to one kinase molecule. We hypothesize that when there is no 14-3-3 binding, both kinases can be blocked by the insertion of the DTS, but this needs to be tested."
The study's computational challenges involved molecular dynamics simulations that modeled the protein at the atomic level, determining the forces of every atom on every other atom for a system of about 200,000 atoms at time steps of two femtoseconds.
"For small systems, we can see what's happening relatively quickly, but for large systems like these, especially large biomolecular systems, these changes happen on like nanosecond timescales, microsecond timescales, or even millisecond timescales," Karandur said.
Karandur and colleagues turned to XSEDE, the NSF-funded Extreme Science and Engineering Discovery Environment, for allocation time on the Stampede2 supercomputer at the Texas Advanced Computing Center (TACC) to do the simulations, as well as the Bridges system at the Pittsburgh Supercomputer Center to investigate other proteins in the pathway. Stampede2's Skylake processor nodes, networked with Intel Omnipath, made quick work of the optimized-for-supercomputers NAMD molecular dynamics simulations.
"Stampede2 runs very, very fast, and it's very efficient. We generated a total of about 1.5 microseconds of trajectories for our systems in about four to six weeks. Whereas, if we ran it on our own in-house cluster it would have taken us months or longer," Karandur said.
About XSEDE, Karandur commented: " I think it's an amazing resource. I've been running simulations starting from when I was a graduate student. XSEDE made it possible for us to access timescales that are biologically relevant. Everything that happens in a cell happens on microsecond timescales, to millisecond timescales, to longer. When I was starting, we could not run this simulation on any system anywhere. I mean, it would have taken five years or more. To be able to do it in weeks and say, okay, we know understand why this is important so we can now start to gain real understanding into how the biology happens, is just amazing," Karandur said.
And there remains a lot to be discovered about B-Raf. It's just one link in the signal chain that governs cell growth and cancer.
"The structure that was resolved in this paper is part of a large, multi-domain system," Karandur explained. "We don't know what this complete protein looks like. We don't see it in the structure. We don't know what its dynamics look like, and how all these other parts of the protein play a role in maintaining the active state or converting it from the inactive state to the active state."
She furthered that as the system gets bigger, the pertinent structural changes happen over longer timescales, and bigger supercomputers are needed to handle the complexity, such as the NSF-funded Frontera supercomputer, also at TACC.
"Frontera is getting there. We're very excited about this. We are in the process of getting an allocation on Frontera," Karandur said.
For non-scientists, this fundamental research could yield insight leading to better drugs for skin cancer.
"The paradoxical activation of Raf kinase by these B-Raf-specific inhibitors turn normal cells to tumors during skin cancer treatment," Kondo said. Understanding the mechanism of this phenomenon will allow us to design better drugs. Hopefully, our study can contribute to the understanding of this step. In addition, we found mutations in this link between the Kinase domain and the 14-3-3 binding element of the B-Raf molecule, which was never shown before. This mutation reduces the activity of B-Raf in the cells. It's also indicating that this part of the kinase domain can be a target point to develop new kinds of B-Raf inhibitors."
Said Karandur: "There's a lot of dynamics happening in the cell. We are, largely because of XSEDE, only starting to be able to look at things like that. Going forward, the only way we can continue to look at things is by using very, very large supercomputers, because the calculations require a lot of computational power. It's really exciting to be able actually to see these things happen and to say, here are how things change at the atomic level; here are these interactions between these two atoms form or break, and that translates into this huge change at the global level in the overall structure of the protein, and how it interacts with other proteins or other molecules in the cell. We're very excited about where it will go in the future."
Source-Eurekalert