Use of special proteins from microbes to pass electrical current into neurons helps in switching brain cells on and off with light pulses as discovered by scientist of Stanford University in 2005
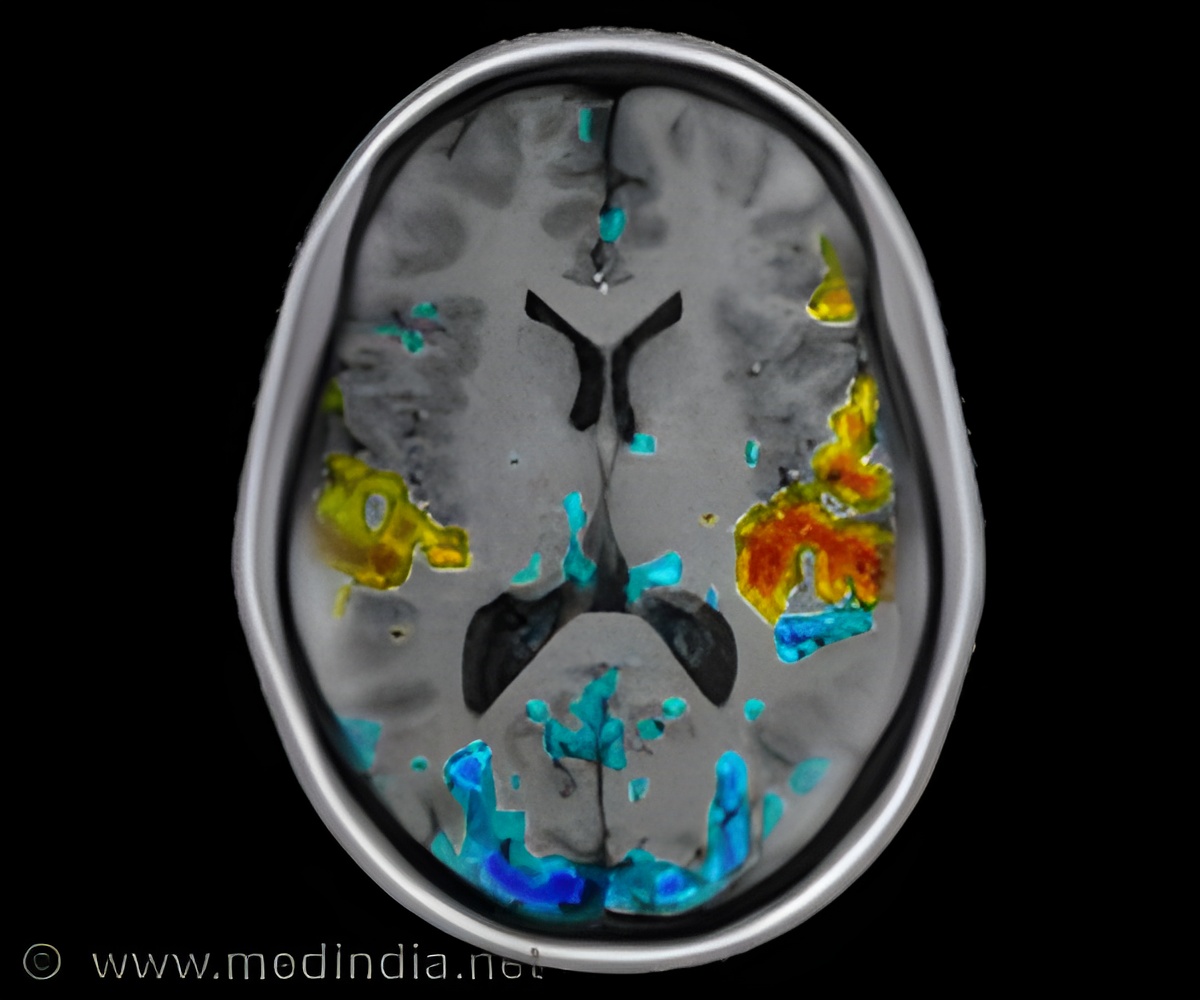
Optogenetics gave researchers a powerful investigational technique to deepen their understanding of biological system design and function in animal models. But first-generation optogenetics had a shortcoming: Its light-sensitive proteins were potent at switching cells on, but less effective at turning them off.
Now in a paper culminating years of effort, Deisseroth's team has re-engineered their light-sensitive proteins to switch cells off far more efficiently than before. The paper will be published April 25 in Science.
"This is something we and others in the field have sought for a very long time," said Deisseroth, senior author of the paper and professor of bioengineering and of psychiatry and behavioral sciences.
Thomas Insel, MD, director of the National Institute of Mental Health, which funded the study, said this improved "off" switch will help researchers to better understand the brain circuits involved in behavior, thinking and emotion.
"This latest discovery by the Deisseroth team is the kind of neurotechnology envisioned by President Obama when he launched the BRAIN Initiative a year ago," Insel said. "It creates a powerful tool that allows neuroscientists to apply a brake in any specific circuit with millisecond precision, beyond the power of any existing technology."
Advertisement
"This is a complex story at the interface of science and engineering," said Deisseroth, who is also the D.H. Chen Professor at Stanford and a Howard Hughes Medical Institute investigator.
Advertisement
As more researchers embraced optogenetics, many discoveries were made with both excitatory and inhibitory opsins. But Deisseroth and other scientists began to notice that stimulation was more effective than inhibition. He therefore sought to lay the groundwork for developing new inhibitory tools by delving more deeply into the mechanism by which the excitatory opsins delivered only positive ions.
Conceptually, the mechanism by which the excitatory opsins operate is simple. Light activation causes the opsin to open a channel through the cell membrane; positive ions then flow through this channel like water through a garden hose. Taking advantage of this channel mechanism, Deisseroth and his collaborators made modified versions of the excitatory opsins in 2008. They called these step-function opsins. A single pulse of light was all it took to flip these switches on and keep the channel open. This allowed the ions to continue flowing, which kept the cell in an excited state even after the light was turned off. The continuous flow of ions enabled by these step-function opsins also made the cell many times more light-sensitive. This allowed scientists to excite neurons deep within the brains of animal models without penetrating the tissue with fiberoptics.
In contrast, none of the inhibitory opsins acted as channels. They were all "pumps" moving only a single ion across the membrane for every incoming photon — acting more like a squirt gun than a garden hose. Each incoming photon was like pulling the trigger to deliver a negative ion. But it took another photon, or trigger pull, to deliver the next negative ion, and so on. This pump mechanism was less efficient than a channel mechanism in terms of ions moved per photon. It was also impossible to engineer the inhibitory opsins to stay open. Therefore it took more intrusive use of light to perform experiments. Finally, the inhibitory pump did not work in the same way as the normal mechanisms that inhibit brain cells. The normal mechanisms make neuron membranes leaky — like water balloons with pinpricks — and more resistant to firing. All these factors made opsin pumps less efficient as inhibitors. Ideally, bioengineers wanted an inhibitory opsin that functioned like a channel.
In a 2012 article in Nature, Deisseroth's team (with collaborator Osamu Nureki, PhD, director of biological sciences at the University of Tokyo) completed the first big step along the path toward improving optogenetic inhibition when they revealed the detailed structure of the on-switch channel protein. This work showed that the amino acids inside the channel pore created a lining of negative charges. This negative lining attracted positive ions to flow through the membrane in order to excite the cell. This discovery pointed to a potential strategy to create an inhibitory channel: bioengineer the opsin to create an inner lining of positive amino acids to draw a flow of negative (inhibitory) ions, such as chloride, into the cell.
From that point, it took roughly another two years for Deisseroth's team, led by Andre Berndt, PhD, and Soo Yeun Lee, PhD, postdoctoral scholars in bioengineering and lead authors of the paper, to complete this process. In order to create this new lining, the Stanford team re-engineered the excitatory channel opsin from their 2012 experiment to change nine of the protein's roughly 300 amino acids. When trigged by light, this newly bioengineered protein now opened a channel lined by more positively charged amino acids, and thereby attracted a flow of negative (chloride) ions to inhibit activity. This created a microbial opsin capable of delivering a powerful channel effect for inhibition.
Finally, fulfilling one of the most anticipated goals of the project, they changed a 10th amino acid, which gave them the ability to keep this new negative channel open — making the negative channel more like the positive channel. As hoped, this created a vastly more light-sensitive inhibitory channel opsin. The targeted neurons remained off for several minutes after a single, brief, blue light pulse, in a manner that was instantaneously reversible with red light. Deisseroth called this new negative channel opsin SwiChR.
As the Stanford team continues a 10-year journey that began with its first microbial opsin experiments in neurons, Deisseroth anticipates that the long-acting and stable responses of SwiChR will open new realms of opportunities for optogenetics.
His enthusiasm was echoed by Merab Kokaia, PhD, a professor at Lund University Hospital in Sweden who has used optogenetics to study epilepsy, among other conditions, in rodent models. Kokaia, who was not involved in the Deisseroth team's new study, noted the new opsin's main advantages: more effective at inhibiting neuronal activity based on chloride conductance, greater sensitivity to light, and the ability to stay open for longer periods and maintain inhibition even without light for longer periods.
"These features could be much more useful for behavioral studies in animals but could also become an effective treatment alternative for neurological conditions where drugs do not work, such as some cases of severe epilepsy and other hyper-excitability disorders," Kokaia said. "The novel approach of channel engineering used by Deisseroth's lab opens unprecedented perspectives for developing new optogenetic tools for system neuroscience studies that will help us to understand better how the brain works."
Charu Ramakrishnan, a research assistant in the Deisseroth lab, also was a co-author of the paper.
Source-Eurekalert